Motor modeling for Vehicle Electrification. Optimizing EV motors, thanks to COMSOL Multiphysics motor modeling capabilities.
Electric motors and drive systems have existed for almost 200 years, and so have electric vehicles. Today, there are many different motor designs available, targeting different markets. Some motors are developed to be inexpensive, some to be efficient, and others powerful. However, never before has there been such a strong incentive to combine all three criteria in one design. The search for clean energy solutions has created a sense of urgency; with a new generation of electric vehicles on the rise and many billions of dollars being poured into electric drivetrain development – by both industry and government stakeholders – there is a strong need for advanced numerical analysis.
Furthermore, there is no single winning design. There are at least a handful of motor types that could claim the throne, and their requirements differ depending on the particular application, whether it is the transport of goods, public traffic, or motor sports, for example. To push the envelope even further, some companies have combined different motor types, like Koenigsegg Automotive AB did recently with its “raxial flux” motor. Finally, the aerospace and marine industries are pushing for electric solutions as well. All in all, there is a vast landscape of options to explore for achieving the best motor design, and 3D motor modeling will be paramount to its success.
Comparing 3D Motor Designs
Extruded 2D
Relatively simple 3D model. Minimal hardware requirements. Very close agreement with 2D models. Helps investigate and validate the basics of 3D modeling (figure 2).
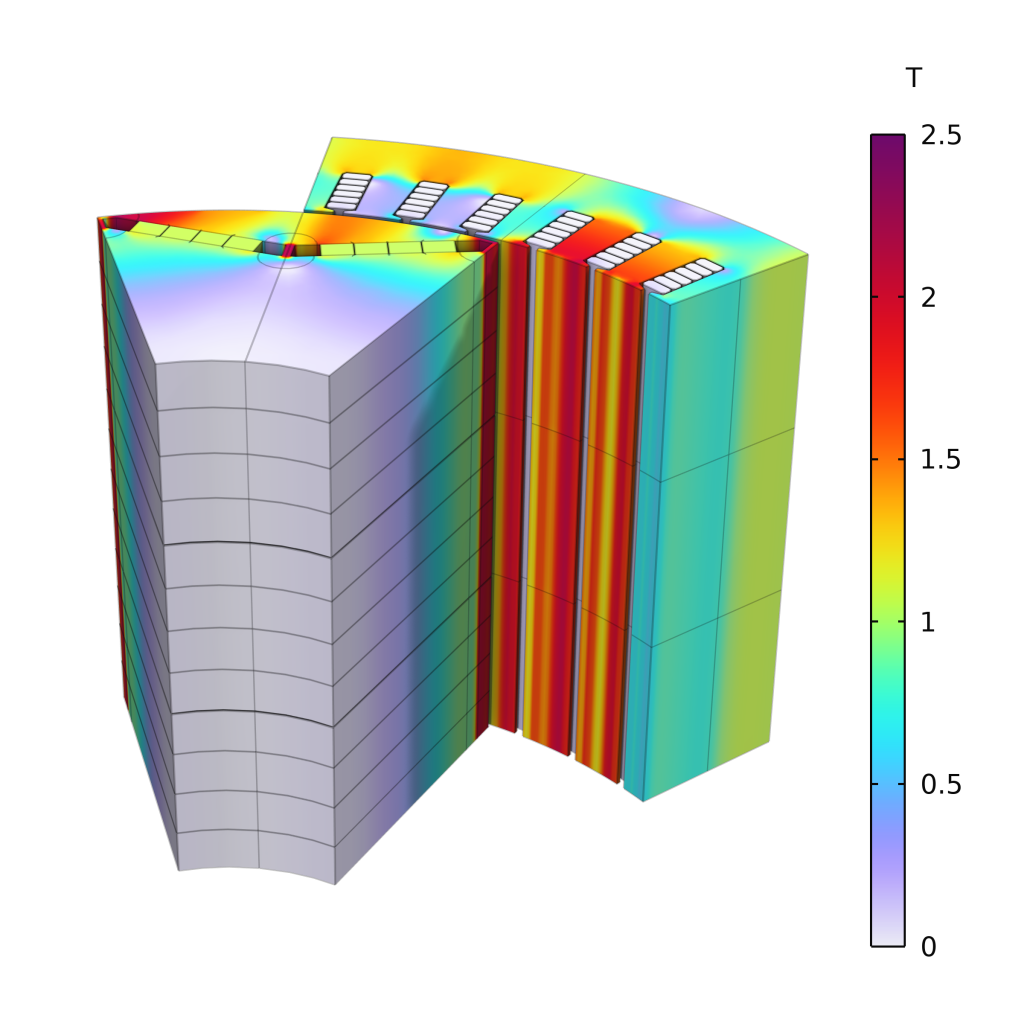
Symmetric 3D
Assumes symmetry at the axis midpoint. (Front and back are solved sequentially.) Moderate hardware requirements. Effective method for investigating the end effects and several skew configurations (figure 3).
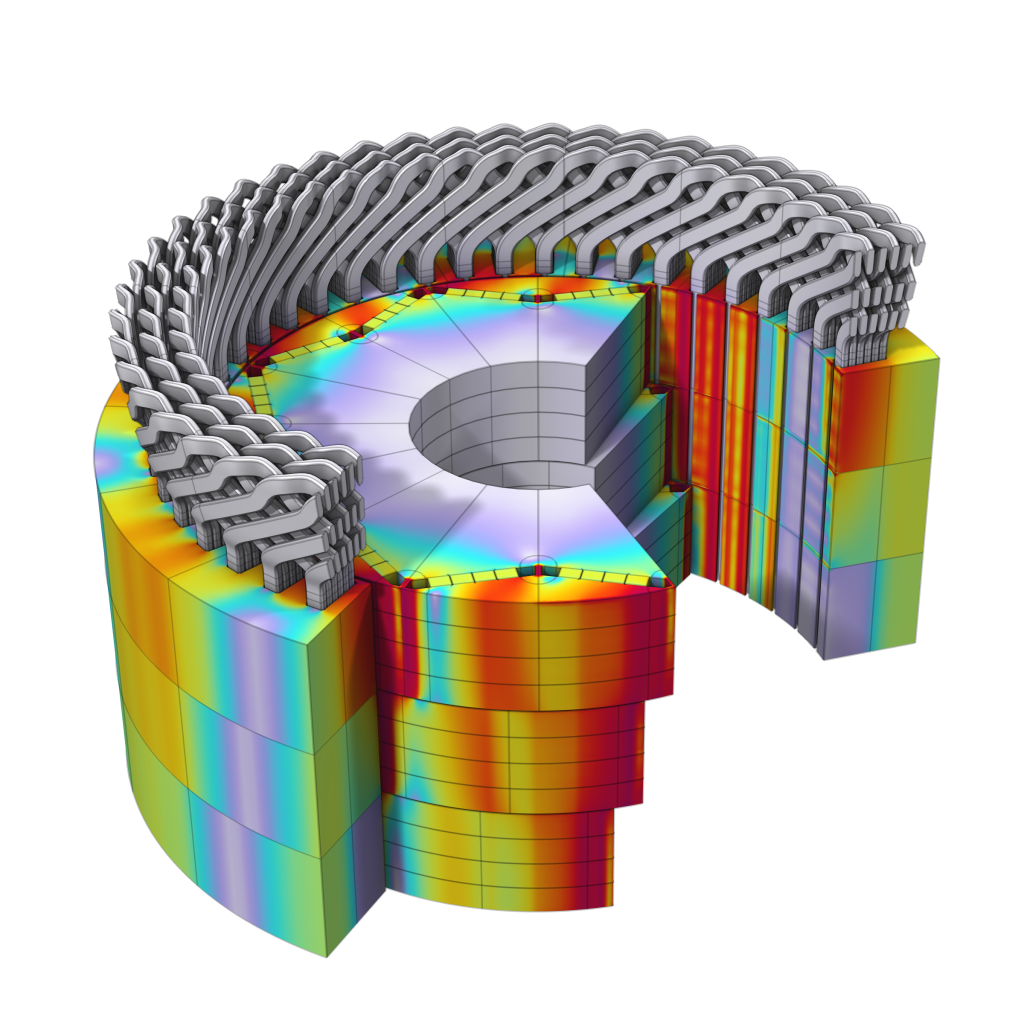
Full-Length 3D
Assumes sector symmetry only. Moderate to substantial hardware requirements. More suitable for complicated skew designs (combined rotor–stator skew) and fully coupled multiphysics phenomena that may break the symmetry. Supports a wide range of configurations to calibrate 2D models (figure 4).
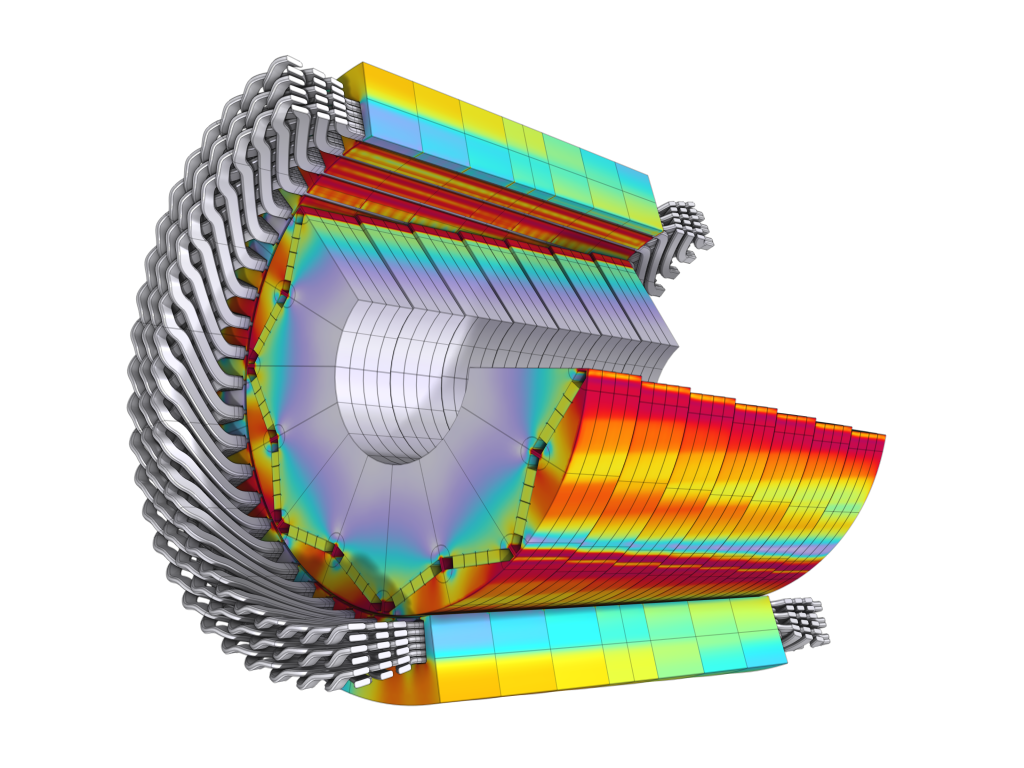
Calibrating 2D Motor Models
Once tested and conditioned properly, 2D and 2.5D motor models can be very useful indeed, especially within the context of topology optimization or parameter sweeping. Many 3D effects can be replicated or approximated with 2D models. This can be done for radial flux machines and, to some extent, axial flux machines too (figure 5).
2D models have a number of blind spots, though. Traditionally, they have had to be calibrated and verified using very costly prototype development cycles. Well-established manufacturers have typically relied on data they have gathered over many years, sticking to familiar designs. Now, with recent advancements in hardware and software development, 3D motor models can close the gap, enabling considerable cost reduction and faster product development.
An important preliminary step is to develop trust in 3D models. This can be done by looking at simple cases for which the 2D solution is known to be exact — like a 3D model that is no more than a simple extrusion. These configurations can be used to tune the 3D meshes and solver settings to find a good compromise between solving speed and accuracy. Comparing with measurements is an option too.
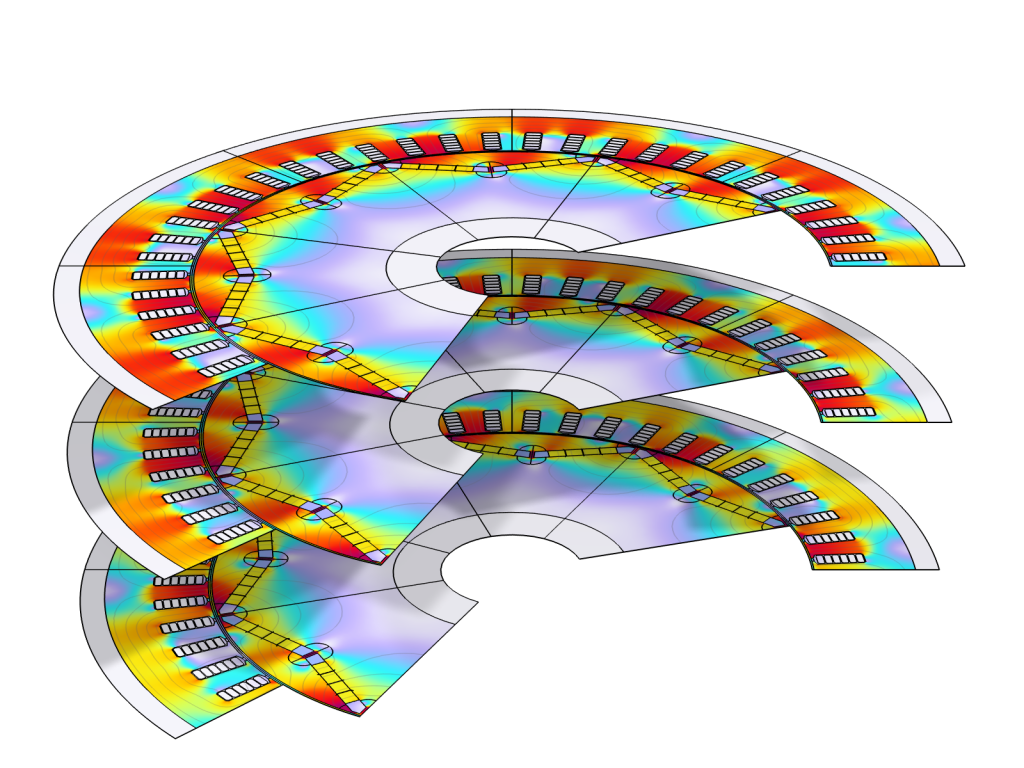
From this point onward, the 3D model can take many forms — with or without skew, with or without end turns, with or without magnet segmentation cuts, and so on — and a comparison between these forms can beused to isolate certain effects. The next step is to determine to what degree they can be replicated in 2D and, if they cannot be, if there is some underlying logic that can be used to augment the 2D models to better reproduce the 3D results — and ultimately, the measurements.
When doing so, the 3D models will be very useful to map the key points of interest: Even if we do not know precisely which materials are going to be used or which thermal conditions are going to apply, we can still investigate which input parameters have a strong influence on the performance of the machine and which can be ignored — or can be used to save costs.
Finally, it is important to keep in mind that the electric motor is a multiphysics machine. Therefore, an optimal design will take into account mechanical, acoustical, and thermal performance as well.
2D vs. 3D Motor Modeling
Historically, 2D motor modeling has been dominant because it has provided sufficient accuracy for very little computational effort. However, in recent years, the demand to fully resolve 3D phenomena has increased. Together with enhanced motor modeling software and increasingly powerful hardware, this demand opens up a whole new market.
Full-fidelity 3D modeling provides valuable insight into many effects that otherwise are difficult to reliably quantify. Some of these can be replicated in 2D, some can be approximated, and some can be compensated for. Using the COMSOL Multiphysics® software, we have investigated a number of these effects, including:
- Fringing effects
- Torque ripple reduction by means of rotor skew, stator skew, or the application of grooves
- Flux leakage between step-skewed rotor segments
- Eddy currents in the magnets and the effect of magnet segmentation cuts for suppressing them
- Resistive effects, inductive effects, and loss in the end turns
- Skin and proximity effects inside and between the turns
- Electric field singularities increasing the risk of electromagnetic breakdown
In addition, there are several multiphysics effects to consider, such as those related to heat transfer, structural mechanics, acoustics, and fluid dynamics.
A move toward 3D motor modeling seems inevitable but should not be seen as a solution on its own. In practice, the best development strategy involves a combination of 2D modeling, 3D modeling, and prototyping. Computationally demanding research such as topology optimization studies will typically still be done using heavily finetuned 2D models before going back to 3D. In this context, the 3D models serve as the missing link between the 2D models and the prototypes: they allow designers to gain a more profound understanding of the machine, help them to better interpret the measurements and calibrate the 2D models, and – most importantly – aid in making better design choices.
3D Motor Modeling Resources
The COMSOL Multiphysics motor modeling capabilities have been extended in recent years, resulting in better performance and usability, and better example models. For free access to a collection of detailed resources on 3D motor modeling, visit: www.comsol.com/model/110261.
COMSOL and COMSOL Multiphysics are registered trademarks of COMSOL AB.
(by Durk De VRies, COMSOL)